Uncovering a comic book
critter's biochemistry
By Sarah Osborne
OTTAWA — "An
amazing miracle! Create instant life."
Preserved in "instant-life crystals,"
sea monkeys were the mainstay of most comic book's back
cover during the 1960s. Flesh-coloured underwater critters,
stick-legged and round-bellied, lounged around the aquarium
floor, their pink plastic castle in the background.
Children who bought sea monkey kits were
told they had "stepped across the threshold of
one of the strange worlds of tomorrow's science... TODAY!"
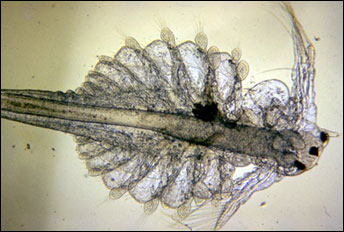
Tomorrow's "strange world" of
sea monkeys – small crustaceans called brine shrimp
Artemia, and not three-horned leisure enthusiasts
– are actually the product of millions of years
of evolution. "Tomorrow's science" has yet
to unlock these tiny, feather-finned animals' secret
of self-preservation.
The sea monkey's ability to create "instant
life crystals" is not just an interesting quirk
of nature. Biochemical and genetic research into the
brine shrimp's method of self-preservation and the proteins
involved may help preserve other animals in the future.
This research will not be done, however, if apathy and
funding concerns take precedent.
Our knowledge of DNA and genetics is
largely due to the sacrifice of millions of fruit flies
and C. elegens worms, while mice, rats and
rabbits and dogs have helped unlock the mysteries of
cancer and diabetes. Yet brine shrimp are still viewed
as that quirky little character from the back of comic
books. Artemia are nourishing little creatures,
used by the bucket in aquaculture, but they are hardly
typical laboratory subjects.
Monkey sea, monkey do
Thomas MacRae, a researcher at Dalhousie
University in Halifax, has studied brine shrimp Artemia
at the cellular level for nearly 30 years. MacRae says
he knows brine shrimp will never be the loyal laboratory
subject, they still hold secrets worth revealing.
"I don't think shrimp will ever replace
Drosophila (fruit flies), mice, rats, or C.
elegens, because those systems are now so well
established for a lot of studies. " says MacRae.
Brine shrimp are native to some of the
harshest ecosystems in the world: inland salt lakes
and salt evaporation pools. They thrive in salt concentrations
other marine animals could not endure; tolerate low
oxygen levels; and survive weather extremes.
Conditions can even become too harsh even
for these small crayfish-like creatures, which measure
no more than 1 centimetre long. If their lake is too
salty, oxygen levels too low, or their food source,
algae, too scarce, brine shrimp will slowly die off.
When this happens, mother Artemia switch over
to a different type of pregnancy. Instead of delivering
baby shrimp into an environment that will kill them,
mothers protect future shrimp by pausing the gestation
process when they reach about 4,000 cells. Baby shrimp
develop not as larvae, but as cysts. Imagine if a mammal
could choose to lay a dehydrated egg halfway through
pregnancy instead of giving birth to a baby.
The cysts are hard, round little capsules
that are nearly indestructible. Once they are expelled
from the mother Artemia, they may sink to the
bottom of the lake, or wash up on shore. The cysts are
in (what MacRae describes as) suspended animation –
or diapause, as biologists say.
Ken Storey, a Carleton University biochemist,
researches animals' different methods of diapause. His
particular field of research is squirrel hibernation.
While Storey reflects the science community's apathy
for brine shrimp ("No one cares about brine shrimp,"
he says), he reluctantly acknowledges brine shrimp's
skill at creating cysts, instead of larvae.
"Brine shrimp are nature's champion's
in that they can encyst, but they are not unique to
that," Storey says. He points to bacteria 250 million
years old encased in salt, which have been revived by
biologists in the lab, and insects in Africa which enter
diapause every year. Nevertheless, both MacRae and Storey
say brine shrimp are perhaps the best animal in the
world at creating these cysts.
Dehydrated and containing only two to
five per cent water, the cysts can survive temperatures
of -273 degrees Celsius or Absolute Zero. This is in
contrast to the human body, which is about 72 per cent
water. If it loses more than 10 per cent of its water,
cells start to die. Hydrated, but without any metabolic
activity, cysts can survive temperatures of –18
degrees Celsius to 40 degrees Celsius. Kept safe from
oxygen, such as in anaerobic mud at the bottom of a
lake, or vacuum-packed, they can survive for years.
Shock to the system
 |
Thomas MacRae studies brine
shrimp at the cellular and molecular level. |
MacRae conducts his research on the cellular
mechanics of the brine shrimp's diapause. Two aspects
are particularly interesting: how the cyst can endure
such severe dehydration yet rehydrate unscathed, and
how the mother brine shrimp actually switches from producing
larvae, to producing cysts.
MacRae says his research lab stumbled,
almost by accident, over a small heat shock protein
that appears to help the cyst endure dehydration and
rehydration.
Heat shock proteins, discovered in fruit
flies in the late 1970s, are also found in every animal
cell including those of humans, except for red blood
cells, which have no nucleus. These proteins are abundant
in the heart muscle, and prevent cataracts in the eye
lens.
Perhaps most importantly, they also act
as 'molecular chaperones,' and are especially critical
in protecting the Artemia cyst from becoming
damaged.
Cells constantly produce proteins to keep
themselves in working order. In order to fulfill their
task, proteins, which are complex chemical structures,
must be folded correctly. When a cell is stressed by
heat, dehydration, or lack of oxygen, the proteins start
to unfold. The small heat shock proteins, such as p26
in Artemia, prevent these everyday proteins
from unfolding irreversibly, and helps refold partially
unfolded proteins.
Imagine a slinky toy that has been stretched
so much it is started to get kinks and lose its spring.
A heat shock protein would be able to see the damage
start, move in to prevent the slinky from uncoiling
more, and even fix any damage. This is the chaperoning
aspect of small heat shock proteins, which keep Artemia's
proteins safe, even when it is dehydrated in cyst form.
"It's the term that we all use, but
its probably better to call these things molecular chaperones,
because heat shock proteins can be induced without heat,
and some are produced just normally. Heat shock protein
was just a name that stuck and it came about because
of how the proteins were discovered," says MacRae
MacRae helped scientists at the University
of California Davis, who had the idea of using small
heat shock proteins – in this case the sugar trehalose
– to freeze-dry and rehydrate viable blood platelets.
The average life of platelet cells is five days at no
less than 20 degrees Celsius. By inserting trehalose
into the platelet cells, they can be dehydrated and
rehydrated successfully. Platelets dried with trehalose
have a shelf life of up to three months.
Treating cells with small heat shock proteins
is one thing, but trying to get mammalian cells to express
Artemia's proteins such as p26 is another challenge,
one that MacRae says his lab is tackling. If successful,
the knowledge could be used to freeze-dry more complex
cells in a way that preserves the cell's nucleus for
cataloguing or future study.
Mixed signals
While p26 protects brine shrimp cysts
while they are dehydrated, MacRae says the actual mechanism
of diapause is still only partly understood. Scientists
do not know how much of the diapause the cysts take
on themselves, and how much is caused by the mother.
"She's responding to some signal
from the environment, and producing a hormone which
has to effect these embryos. It could be that these
decisions are made very early, maybe even before the
eggs are fully developed and ready for fertilization,"
says MacRae.
That is not the only mystery of the brine
shrimp's diapause. The cysts have to dehydrate in order
to be 'reactivated' from their non-metabolic state,
for instance, but nobody knows exactly why. There is
speculation it has to do, yet again, with small heat
shock proteins.
MacRae says understanding the mechanics
of diapause may have an impact on understanding aging,
but that aquaculture companies are interested as well.
Artemia are already bred for higher yields
and longer storage, but they can be farmed even more
efficiently once their diapause is fully understood.
Storey says the main impediment to brine
shrimp research is that Artemia are not useful
enough to entice researchers to bother sequencing the
genome, which can cost millions of dollars.
"Brine shrimp are not going to help
us make freeze-dried soldiers," he says. "Brine
shrimp have a very complicated genome that nobody cares
about."
Yet having a complete genome can make
researching an organism's biochemistry markedly easier.
The technology to sequence genomes is still so expensive
and new, that only organisms of special interest to
researchers are sequenced – such as C. elegens,
mice and rats, the chicken, the potato, and about 14
different types of fruit fly. Storey says when scientists
eventually get around to sequencing a crustacean, it
will probably be a more marketable one, such as the
lobster.
MacRae says he and some other North American
colleagues have planned a trip to Beijing in April,
where they want to convince Chinese scientists to take
on the job of sequencing the brine shrimp genome.
Human connection?
The lack of interest in Artemia
biochemistry is also reflected in the difficulties MacRae
faces trying to get funding. Although MacRae's work
is funded by the Canadian Institute of Health Research,
the Nova Scotia Health Research Foundation, and the
Heart and Stroke Foundation, it is hard sometimes to
show how his research applies directly to human health.
"We're interested in the biology
of the organism because its an interesting organism,
it's an unusual organism to look at, but we're also
trying to apply it to a medical situation or aquaculture...
And of course there are many reasons to do that. One,
you feel more useful, but two, it's much easier to get
money," says MacRae.
"We're studying brine shrimp, which
is a semi-obscure organism, and people will ask me,
'well why would you want to be studying that when you
could be studying cancer?' My answer is generally, 'well
I am studying cancer, because I'm studying cellular
biology and chemical biology.' "
Storey says that as long as conducting
research into genetics remains expensive, organizations
are going to want to see a direct human application
to the research they fund.
"I'm not saying it's right, I'm
just saying that's the way it is. And right now, the
funding gods are smiling on [this] way of seeing things,"
says Storey.
Ultimately, the proteins MacRae studies
are present in humans, and often perform similar functions,
acting as molecular chaperones, and preparing the cell
membrane to grow with the cell. MacRae says this makes
brine shrimp ideal to serve as a "system model,"
a sort of square one, in the particular functions it
excels at, which can then illuminate how the human equivalent
works. This is also his approach when requesting funding.
"It's a bit self-serving, but at
the same time we are encouraged that whatever our obsessions
are, to try and apply them to something useful where
everybody else gains, which I think is fair," says
MacRae.
"When you work with brine shrimp,
it's not perceived so directly as being medical, but
if I'm studying a protein which is found in your eye,
and I can decide or determine things about my protein,
then you can then extrapolate it to your system."
In short, by studying how a protein like
p26 works in brine shrimp, scientists have helped discover
how p26 may prevent cataracts in the human eye.
MacRae says his lab's work on brine shrimp
is fundamental biochemistry and molecular biology, and
it adds to the basic literature in those areas. He says
studying the basic biology of an organism that is on
the sidelines of genetic research can still be useful.
MacRae says science shouldn't always be
approached for its direct human impact. "If you
want to count the bristles on the legs of an insect,
which at first sight doesn't seem to have much use,
I'm happy for you to do that, because you don't know
what's going to come out of it down the road. And that's
more or less what's happening with what we're doing
now," he says.
|