Super Kamiokande
The SNO isn't the sole experiment credited with solving the solar neutrino mystery.
A Japanese-American experiment known as the Super Kamiokande, detects all three types of neutrinos.
Since SNO detects only electron neutrinos, if only electron neutrinos travel from the sun to the Earth, then SNO and Super Kamiokande would measure the same number of neutrinos.
If some solar neutrinos were muon or tau, then Super Kamiokande would measure a larger number of neutrinos.
And this is exactly what happened.
|
Deep inside a hot, humid nickel mine near Sudbury, Ont., Alain Bellerive and a research team from Carleton University are trying to solve a fascinating case of multiple personality disorder.
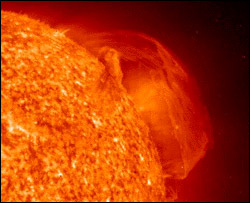 |
Neutrinos may be detected in a solar flair such as the one pictured above.
© Sudbury Neutrino Observatory, Carleton University Site |
But Bellerive is no expert therapist, psychologist or psychiatrist studying the human mind. He is a physicist searching for clues about subatomic particles called neutrinos.
Neutrinos are tiny bits of the sun so small that 100 billion of them are passing through your thumbnail at this very second.
Detecting particles that small sounds impossible. But that's just what Bellerive and his team are doing at the Sudbury Neutrino Observatory (SNO) in an attempt to explain the multiple personalities of neutrinos. They are part of an international collaboration made up of 113 scientists from 11 universities and laboratories in Canada, the United States and the United Kingdom.
Last June, they released groundbreaking results from the first phase of the experiment that helped solve a 30-year-old mystery that has puzzled scientists for decades.
Neutrinos are ghostly subatomic particles, produced by nuclear reactions in the core of the sun. They travel from the core of the sun to the Earth. There are three kinds of neutrinos: electron neutrinos, tau neutrinos and muon neutrinos. Physicists like to refer to these three types as "flavours."
The sun only produces electron neutrinos but some other "flavours" of neutrinos also reach the Earth.
For years, physicists thought they knew how many neutrinos ought to be reaching the Earth, based on laboratory measurements. But every time they tried to count them, they came up with a much smaller number than their models predicted. This was known as the Solar Neutrino Problem. There were two possible explanations: that their calculations were wrong, or something was happening to the neutrinos on their way to the Earth.
The most logical answer, which was proven by the release of the SNO results on June 18, 2001, was the latter: neutrinos have multiple personalities. Electron neutrinos have been arriving on Earth disguised as muon or tau neutrinos.
Somewhere during their eight-minute journey from the core of the sun to the Earth, neutrinos spontaneously switch from being electron-flavoured to one of the other two flavours, tau or muon. Existing detectors could not recognize these neutrino oscillations.
Physicists had been coming up with a smaller number of neutrinos than they predicted, because they were not counting the other two types. If they include all three types of neutrinos, the numbers add up.
In other words, scientists have been trying to eat only the chocolate ice cream in a bowl of Neapolitan ice cream. But they have found that they're only getting about a third of the total amount of ice cream they should be getting. The problem was that they hadn't taken into account the fact that there are also vanilla and strawberry flavoured ice cream as well.
To illustrate another way, even if there are many possible shades of orange that can be mixed from two basic colours like red and yellow, all shades remain intrinsic to the basic mix. A single neutrino still embodies all three "flavours" or shades of orange.
But scientists working on the SNO experiment have yet to distinguish between the muon and tau flavours.
"We can only really make two direct measurements, the number of electron neutrinos, and the total number of neutrinos interacting with our detector," says Darren Grant, a doctoral student working with the Carleton SNO team. "It is possible to use neutrino oscillation theory to place limits on which type of neutrinos we might be seeing and make a statement about what type of neutrino the electron neutrinos are oscillating into, but that will come later in the analysis than what we are currently at."
Neutrinos have no electrical charge and interact very weakly with other particles. Most neutrinos pass right through the Earth without ever mingling with a single atom of it.
The tools used to detect these evasive particles are as colossal as the neutrino is minute.
The Sudbury Neutrino Observatory is located two kilometres underground in an old nickel mine in Creighton near Sudbury. It is far away from the Earth's surface in order to block cosmic rays that might make it difficult to distinguish the subtle neutrinos.
The detector inside the mine is about the size of a
10-storey building. In contrast to its surroundings, the environment inside is so clean that it contains less than a teaspoon of dust.
A 12-metre-diameter acrylic sphere, containing 1,000 tonnes of water, is immersed in a 30-metre barrel-shaped cavity. Each time a neutrino comes in contact with this water, a reaction occurs producing a flash of dim blue light called Cherenkov radiation.
David Sinclair, another member of the Carleton SNO team, illustrates it like a scene from the popular animation, The Simpsons.
"If you ever go to a nuclear reactor site, they'll have a pool where they keep the old reactor fuel and if you look into that you'll see it glowing blue and that's because this Cherenkov radiation tends to be in the blue end of the spectrum," he says.
The neutrino reacts with the water to produce a little flash of light.
"It's not a very bright flash. It's about as bright as if you were looking at a candle and the candle is on the moon," says Sinclair.
The sphere containing the water is surrounded by 10,000 photo-multiplier tubes used to detect the flashes of light produced by neutrinos.
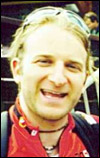
Alain Bellerive
Alain Bellerive, appointed Canada Research Chair in experimental particle physics at Carleton University in Ottawa, is the newest member of the team from Carleton University working on the SNO experiment.
"When I was an undergraduate student, which was back in 1991, I told myself one day I would really like to be a professor in physics and do research in particle physics. So last year when I was awarded this position it was a real honour," he says.
After completing his PhD at Cornell University in the U.S., he worked in Switzerland for almost four years before making the decision to return to Canada, despite lucrative offers for positions in the U.S.
"Carleton University has a really strong background in particle physics but at the end it's really the people that I work with that make the difference. "
|
"Photo-multiplier tubes look like big light bulbs. They're eight inches in diameter, and these can detect single photons of light," says Sinclair.
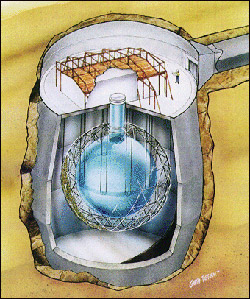 |
The SNO detector is about the size of a 10-storey building.
© Sudbury Neutrino Observatory, Carleton University Site |
The experiment also requires a special kind of water called deuterium.
Regular water is made of two hydrogen atoms and an oxygen atom. Deuterium, or "heavy" water also has a neutron.
When an electron neutrino collides with the heavy water, a reaction between the neutrino and the neutron in the deuterium occurs, transforming the neutron in the deuterium to a proton, and the neutrino to an electron. The electron emits Cherenkov radiation and the photo-multiplier tubes
detect this light.
"Knowing that we can use these great apparatuses was what really triggered me," says
Bellerive.
"What attracted me were this grandiose fact and the meaning to get to the smallest scale as possible to see not only the proton and neutron, which in high school we were taught were elementary particles, but to try to still divide the quarks and leptons and try to understand the foundation of matter of the smallest scale. It's like this cathedral of science and to probe the really small you have to get those cathedrals."
But like cathedrals, immense, intricate apparatuses like the Sudbury Neutrino Observatory are expensive to build.
SNO started in 1990, was completed in 1998 at the cost of $73 million Cdn and the first measurements began the next year.
The cost of construction was funded by three major grant agencies from each of the three collaborating parties: Canada, the U.S. and the U.K.
Sinclair has recently submitted a proposal for about $30 million from a new funding agency set up by the Canadian federal government.
"The goal is to expand the underground and surface facilities and make our 'little' lab into an international underground experiment hall," says Grant.
Meanwhile, the SNO experiment launches into its second phase.
"We've added NaCl, better known as table salt, to the heavy water, and this increases the efficiency of the detectors. The added salt will triple the number of Cherenkov flashes produced from neutrino collision," says Bellerive.
They are expecting results as early as the beginning of the summer of 2002.
"We're making a real leap with the salt data. The solar neutrino problem has been around for 30 years and we have essentially solved the problem. To say our model of how the sun burns seems to be right and what comes out may be that neutrinos oscillate," says Bellerive.
The SNO experiment has other importance as well.
Understanding neutrinos helps us to understand the basic structure of matter, Bellerive says. It helps us to determine the make-up of the universe. It will help us to solve questions like "what is dark matter?"
If neutrinos are oscillating, if they have multiple personalities, then according to the laws of physics they must have mass.
"Suddenly there is mass difference between those particles and therefore you have to somehow remodel the so-called Standard Model, which has described elementary particles for more than 20 to 30 years," says Bellerive.
Even with the understanding that neutrinos contribute to the mass of the universe, their mass is not significant enough that we can rule out neutrinos as making up that unknown realm of dark matter. For some, it is their passion for knowledge that drives their dedication to the long search for clues about these mysterious particles.
"It's just that opportunity to gain some deeper sense of understanding of what is happening in the universe. That's what does it," says Grant.
"You know when you're small and you're in the countryside and you look up and you see all those stars and you think, how does that work? And you think it would be so cool to figure that out, so you study hard and an opportunity like SNO presents itself this beautiful experiment, incredibly designed, an engineering marvel and maybe just, maybe you get to figure something like this out which people have not been able to figure out for [over] 30 years. You get to be a part of that and along the way you get some of these questions answered."
The team at Carleton University has played an important part in the experiments at the Sudbury Neutrino Observatory. They are eagerly anticipating the results of the second phase of the experiment.
"By summer I hope I will have some results and then by next Christmas, publish that result and make a statement about neutrino oscillation with salt," says Bellerive.
|